The Rocket That Could Take Humans to Mars Has Been 50 Years in the Making
Most of today’s rockets generate immense energy from chemical reactions. There’s another way — and the technology has been with us for a generation.
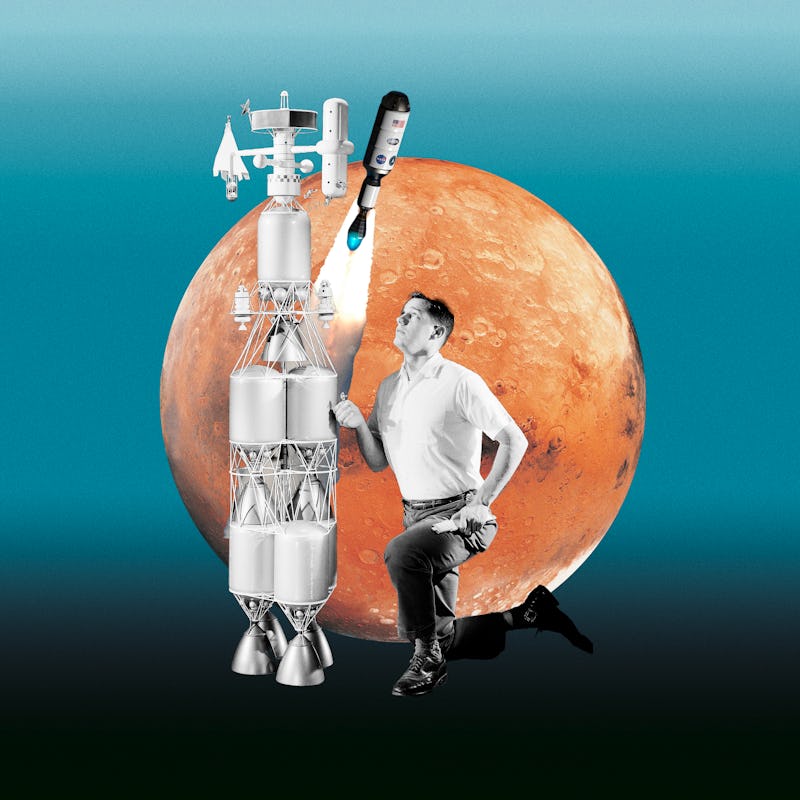
In 2025, if all goes according to plan, NASA will launch the first-ever nuclear fission-powered rocket into space.
The Demonstration Rocket for Agile Cislunar Operations (DRACO) won’t go very far. It will only reach an orbit above Earth, the distance of most satellites. But if DRACO is successful, its successors could set out for Mars or the outer solar system. Nuclear fission can reach Mars or the outer solar system far more quickly and efficiently than today’s rockets. In fact, nuclear fission might be the only way humans ever make it to the Red Planet.
“The final goal is to get to Mars,” Fatima Ebrahimi, a physicist at Princeton Plasma Physics Laboratory, tells Inverse. “You really need nuclear for that.”
Reviving a half-century-old dream
Most of today’s rockets are chemical rockets, generating immense energy from chemical reactions. If you’ve ever seen a chemical rocket launch, you know how spectacular it can be. “Chemical rockets have the advantage of producing large thrust — they are very powerful,” Ebrahimi says. “But they cannot go very far.”
If we want to get to Mars with today’s chemical rockets, we have to take advantage of narrow windows when our planets’ orbits align, which come just once every 26 months. To reach the outer solar system with those same rockets, we usually have to undertake an even more complicated path, dipping closer to the Sun and harnessing the gravity of a planet like Venus to pick up sufficient speed. It’s also quite time-consuming. To reach Jupiter in the early 2030s, for example, we would need to launch today.
Enter nuclear energy.
“The final goal is to get to Mars ... You really need nuclear for that.”
If the phrase “nuclear rockets” sounds old fashioned, you aren’t wrong. Nuclear rockets were an invention of the 20th century, not the 21st. But in staking a nuclear-powered future, the American space program is reviving a dream that is over half a century old.
Engineers have been tinkering with nuclear rockets since the dawn of the Space Age. In the 1950s, when nuclear energy was new and exciting, the U.S. Air Force and Atomic Energy Commission teamed up to develop Project Rover, a prototype nuclear engine that could power a missile.
Then came NASA, which took over the program, ditched the missiles, and transformed the tests into a dedicated program to get a nuclear engine into space: Nuclear Engine for Rocket Vehicle Application (NERVA). In the sands of a nuclear test site in Nevada, NERVA fine-tuned a sequence of test-fired engines. At least on the ground, the tests were successful.
A 1964 nuclear rocket prototype where the crew would be housed in the upper stage with a heavily shielded bulkhead separating them from the engines.
The United States wasn’t alone in taking nuclear rockets for a spin. In 1965, the Soviet Union began crafting its own prototype nuclear rocket and kept up the research for decades. Well into the 1980s, Soviet engineers conducted full-scale trials of an engine they called RD-0410 at the Semipalatinsk nuclear testing ground in northeast Kazakhstan. Nevertheless, RD-0410 never fully took flight.
NASA higher-ups wanted to ensure NERVA would not suffer such a fate. Documents from NERVA’s golden age in the mid-1960s label nuclear rockets as a key part of NASA’s future. By then, planners had already drawn up NERVA-powered mission proposals that would make Apollo’s great leaps look like puddle-jumps. Nuclear cargo ferries to moon bases in the 1970s! Regular flights to Mars and back by the 1980s!
As you might imagine, those grand designs never came to pass. After Apollo 11 did its job, pressures from the Nixon administration and budget cuts shifted NASA’s focus to near-Earth projects like Skylab and the Space Shuttle.
A 1970 drawing of the NERVA nuclear rocket engine.
Even though NERVA had political support and over $1 billion of investment, the project didn’t have a place in this new NASA era and was mothballed in 1973 before ever entering space. But even as budget cuts doomed Project NERVA to fade away into the desert — and even as nuclear power, in general, gained a fallout-stained reputation thanks to Three Mile Island, Chernobyl, and the simultaneous rise of an anti-nuclear movement — the idea of nuclear rockets never truly disappeared.
The Return of the Nuclear Rocket
For the pyrotechnic-minded, the words “nuclear rocket” might conjure images of something like Project Orion: a craft that would push itself along by triggering nuclear detonations behind it. In reality, nuclear propulsion is a little more mundane (and, certainly, more compliant with international bans on nuclear explosions in outer space).
The nuclear rockets that engineers propose — to be more specific, what they call a nuclear thermal rocket (NTR) — replace chemical reactions with a fission reactor.
NTR designs vary, but most are built around two key components: the reactor itself and a superheated fluid, typically liquid hydrogen. A turbopump pipes the hydrogen through the reactor, which superheats the liquid into searing gas. In turn, the gas escapes out an attached nozzle, creating thrust. The hotter the rocket can heat the fuel, the more thrust it can produce.
Artist concept of Demonstration for Rocket to Agile Cislunar Operations (DRACO) spacecraft, which will demonstrate a nuclear thermal rocket engine. Nuclear thermal propulsion technology could be used for future NASA crewed missions to Mars.
To the untrained eye, an NTR engine wouldn’t look obviously different from a chemical rocket. Even from the rocket builder’s eye, many of an NTR engine’s components — the valves, the nozzles, the hydrogen that must be kept at cryogenic temperatures — have places aboard a chemical rocket.
Where nuclear rockets shine instead is in their efficiency, what rocket scientists call specific impulse. In other words, how much an engine can boost its spacecraft with the same amount of fuel. NTR engines have two to three times more specific impulses than their chemical counterparts. Once outside Earth’s gravity, nuclear-powered craft can travel far more quickly than chemical craft.
Again, if we wanted to reach Mars using the best chemical rockets we have available, it would be very difficult, with available launch times of just once every 26 months. NTR engines promise to drastically slash those times. Because they can accelerate spacecraft to higher speeds, they open up far more opportunities for flights to other planets that don’t require complex gravitational dances.
“It gives me the ability to abort my mission and return home — not all the time, but for a much longer part of my mission,” Kurt Polzin, chief engineer for space nuclear propulsion at NASA’s Marshall Space Flight Center, tells Inverse.
NTR proponents like Polzin believe that isn’t the only reason a nuclear-powered mission will be fundamentally safer than one with today’s rockets. A shorter journey means astronauts can carry fewer supplies, and their bodies won’t absorb as much ionizing radiation en route.
“If we can improve crew safety, I think we would consider that what we’ve done has been very successful,” Polzin says.
Getting Out of Earth’s Orbit and Onto the Moon
In the 1980s and 1990s, nuclear rockets gathered dust, and most scientists overlooked them. Nuclear rocket designs became the province of secretive U.S. military projects like the Strategic Defense Initiative (the so-called “Star Wars”) Project Timber Wind, which tried to return the technology to its destructive missile roots.
But by the 2010s, a new generation of researchers had taken hold, and NASA’s priorities again began to shift away from Earth orbit. NASA engineers once more dared to dream up plans for nuclear-powered Mars missions.
Within a few short years, it also became clear that the Artemis Program — NASA’s ambitious push to establish a permanent base on the Moon, which could serve as a stopover for human missions to Mars — was actually going to happen. Funding began to follow. In 2019, the U.S. Congress allocated more than $100 million to NTR research.
Dale Thomas, a propulsion engineer at the University of Alabama in Huntsville whose research focuses on NTR engines, thinks there’s one big, Earth-bound factor contributing to the sudden rise in interest in nuclear power. “[Climate change] has caused people to rethink their position on nuclear power in general as a non-greenhouse gas emitting technology,” Thomas tells Inverse. He says shifts in attitudes, which resulted in new nuclear power plants and delays of plans to close old ones, extend to space travel as well.
Thomas, for his part, hopes that studying how to build better NTR engines — building reactors that can stand up to the intense pressures and temperatures of a reactor — can translate into building better reactors down on Earth.
The largest challenge, Thomas says, is “convincing NASA mission planners that they can count on nuclear thermal propulsion in their mission designs.”
That is one goal of DRACO, the mission that plans to launch the first nuclear-powered rocket, picking up where NERVA left off half a century ago.
DRACO will launch atop a standard chemical rocket, partly because chemical rockets are currently more powerful and partly to minimize the risk of a nuclear accident. That rocket will take DRACO up to a relatively high orbit above Earth — somewhere between 435 and 1,240 miles (700 and 2,000 kilometers) — where engineers will take DRACO’s nuclear drive through preliminary tests in a space environment.
“We’ve never done this before ... we’re going to learn how to do it … we’re going to take that and pay it forward to the next system.”
DRACO inherits NERVA’s legacy and research results, but there are a few differences between the two. For one, where NERVA used weapons-grade uranium, which is over 90 percent uranium-235, DRACO uses what is called high-assay low-enriched uranium (HALEU), which is more like 10 to 20 percent uranium-235. This brings DRACO closer to the uranium that’s used in the civilian nuclear power sector. While HALEU does generate less power, acquiring a license to play with it in the U.S. is far easier and far cheaper than getting permission for the higher-grade stuff.
Additionally, part of the reason DRACO’s builders are so eager to test it in space is that, with NERVA’s facilities long abandoned, the original testing grounds are no longer usable. In that sense, it’s already getting off the ground far more quickly than NERVA ever could.
“I just hope that the mission is successful as planned,” Thomas says. “That is, a successful flight demonstration of a nuclear-thermal-propulsion-powered spacecraft.”
DRACO is a prototype. Much of the test’s value will be in letting NASA’s engineers gain practical knowledge: practicing getting DRACO into space in the first place, understanding the quirks of an NTR engine in operation, and creating flight checklists for a type of engine they have never flown.
“We’ve never done this before,” Polzin says. “We’re going to learn how to do it … we’re going to take that and pay it forward to the next system.”
There is still a great deal of work to be done. For instance, Polzin says that engineers need to push NTR engines to even higher temperatures than DRACO. But Polzin firmly believes that “next system” — or the one after it, or so on — will be what finally pushes astronauts to Mars.
NTR may be just the beginning of a growing category of what some scientists and engineers call “advanced propulsion” — or propulsion that doesn’t rely on standard chemical reactions.
Probes to destinations near Earth rely on electric ion propulsion, which uses electric current to accelerate xenon or krypton ions out of a thruster to push it forward. In fact, future craft may generate that current with nuclear fission. More hypothetically, engineers have proposed craft that very literally sail through the solar system with solar sails, which would catch the sun’s light as an oceanic ship’s sails catch the winds. Meanwhile, Princeton’s Ebrahimi is one of a handful of scientists working on engines powered by a different sort of nuclear physics: fusion.
There is no reason we have to only power spacecraft with one type of engine. The future of space travel might be all of these options existing in tandem, each one specialized for a specific purpose.
This article was originally published on