Ghost Particles Could Help Untangle Quantum Gravity
Physicists look to neutrinos for clues about how gravity works at tiny scales.
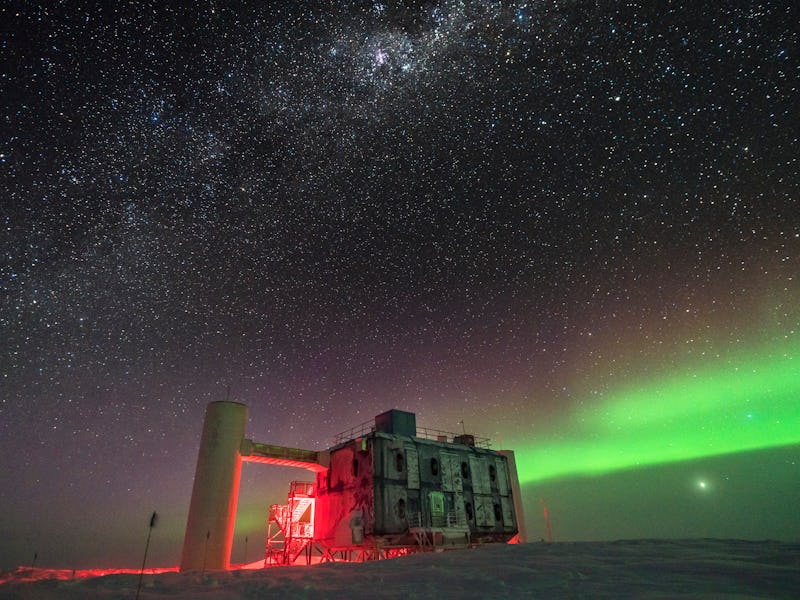
Sensors buried deep in the Antarctic ice may help physicists figure out how gravity works in the quantum realm, the smallest scale of existence.
A team of physicists says they’ve figured out how to use a sensor-laden patch of ice near the South Pole to measure tiny changes in particles called neutrinos as they pass through space. Those changes could help reveal how gravity affects matter on the scale of subatomic particles like neutrinos. If they’re right, it could be a step toward resolving a problem physicists have wrangled with for decades.
Physicist Tom Studdard of the University of Copenhagen and his colleagues published their work in the journal Nature Physics.
The IceCube lab stands near the Amundsen-Scott South Pole Station in Antarctica. Beneath the surface, neutrino detectors embedded in a cubic kilometer of ice wait for a neutrino to streak past.
Quantum physics on ice
Studdard and his colleagues studied data from the IceCube Neutrino Observatory in Antarctica, whose sensors have recorded the passage of around 300,000 neutrinos so far. The physicists wanted to know whether they could measure tiny, subtle changes in the neutrinos’ properties, which could be caused by an elusive force called quantum gravity. Stuttard and his colleagues showed that their method for measuring those small changes worked, but they need more data to find what they are looking for.
The universe as we know it works according to a set of rules described by physicist Albert Einstein in his theory of general relativity. Those rules explain everything from why a dropped pen falls to the floor, to why galaxy clusters can become cosmic magnifying lenses. But if you zoom in to very tiny scales — the realm of subatomic particles like electrons, quarks, and neutrinos — Einstein’s rules don’t seem to apply anymore. At the smallest scales, the universe runs on a different set of rules called quantum mechanics.
Somewhere, there’s an exact scale at which the rules change over from general relativity to quantum mechanics, and somewhere, there’s a way to connect the two sets of rules. That’s the kind of problem that has kept generations of physicists awake at night. Right now, there isn’t a good description of how (or if) gravity works at the tiny scales of quantum mechanics. Studdard and his colleagues hope neutrinos can help them learn those rules.
But they’re going to need a lot more neutrinos.
Shape-shifting ghost particles
Neutrinos are like tiny ghosts; they have no electrical charge and almost no mass, so they barely interact with the universe around them. Like ghosts, they can move through walls, mountains, or even entire planets, undisturbed and often undetected. Most of the neutrinos IceCube’s sensors detect have passed through the roughly 7,800-mile diameter of Earth to arrive in the Antarctic ice.
Even IceCube doesn’t really detect neutrinos; instead, its sensors (thousands of them, embedded throughout a cubic kilometer of deep, ancient ice) measure the quick flash of radiation that happens when a neutrino bumps into an atom. That radiation holds clues about the neutrino’s energy and the direction it came from. Stuttard and his colleagues hoped that information could also reveal whether certain properties had changed slightly during the neutrino’s journey.
Here’s where it gets weird. (No, it wasn’t weird before. You haven’t seen weird yet.)
Neutrinos come in three types, or flavors: electron neutrinos, muon neutrinos, and tau neutrinos. Those flavors are named for the types of subatomic particles that tend to be spawned when the neutrino finally collides with an atom. And as a neutrino flies through space, it changes flavors constantly.
“Imagine purchasing a carton of chocolate ice cream at the store, driving home, and opening it only to find it was vanilla! So you put a scoop of vanilla in your bowl and walk into the other room to eat it, where you are surprised to find it is now strawberry,” explains the Fermi National Laboratory on its website. “That’s what happens with neutrinos.”
However, those changes aren’t random; physicists can generally predict how far a neutrino should travel between flavor changes. Stuttard and his colleagues were looking for deviations from that predictable pattern (which is called quantum coherence). If those deviations happened, they had to be caused by something actually having an effect on the neutrino — something like a version of gravity that works at the quantum scale.
A long way to go
Stuttard and his colleagues didn’t measure any quantum-gravity-induced changes in their neutrinos, but they did show that it’s possible to measure whether a neutrino’s quantum coherence has been tampered with mid-flight.
Most of the neutrinos that IceCube has measured so far formed in Earth’s atmosphere when high-energy particles from space collided with atoms in the atmosphere. At most, those atmospheric neutrinos had traveled about 7,800 miles through Earth to reach IceCube. That’s not very far in the cosmic scheme of things.
“Apparently a much longer distance is needed for quantum gravity to make an impact if it exists,” says Studdard in a recent statement.
And that means physicists are going to need data from many, many neutrinos that began their journeys in distant space, traveling billions of light years to reach Earth. Those neutrinos are rarer and harder to detect than atmospheric neutrinos, but Studdard and his colleagues are optimistic.
“With future measurements with astrophysical neutrinos, as well as more precise detectors being built in the coming decade, we hope to finally answer this fundamental question,” says Studdard.