Scientists Claim a New Invention Could Make Nuclear Fusion a Practical Reality
But it isn’t clear if it works.
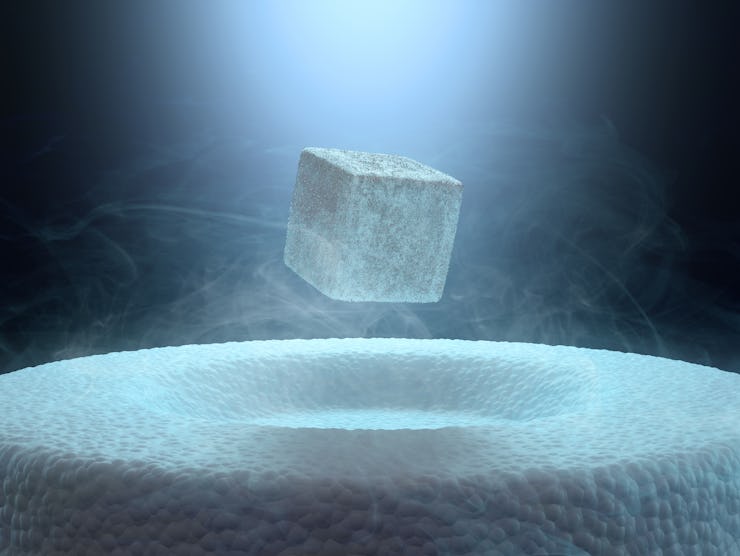
Superconductors excel at, you guessed it, conducting electricity — these materials help a current flow without any resistance, a critical feature for powerful tech such as MRI machines and levitating trains. One day, they could even make more efficient energy grids, faster electronics, and even practical nuclear fusion reactors possible.
But today’s superconductors are far from perfect. For over a century, all known superconducting materials worked only at super-cold subzero temperatures, which can prove inconvenient. In 2020, scientists revealed what they claimed was the world’s first room-temperature superconductor, but it only worked at extremely high pressures.
Now, in a new study published in the journal Nature, researchers from the University of Rochester in New York say their new room-temperature superconductor works at pressures low enough for practical applications. But in recent years, these scientists have charged up some drama.
Scientists created the Baseball II superconducting magnet for early fusion experiments in the 1970s.
Go with the flow
Regular electrical conductors all resist electron flow to some degree, resulting in lost energy. Meanwhile, superconductors conduct electricity with zero resistance, potentially allowing for far more efficient power grids and electronics. "We can envision this applied to commonly used devices so laptops don’t heat up," Ranga Dias, a physicist at the University of Rochester in New York and senior author of the new study, tells Inverse.
Today, superconducting wires made of metals such as titanium and niobium conduct much larger currents than ordinary wires. They can even generate the powerful magnetic fields that enable high-speed floating trains, MRI scanners, and particle accelerators. Eventually, they could be used in the now-elusive nuclear fusion reactors.
The recent feat has been over a century in the making: Superconductivity was first discovered in 1911. At the time it only worked at temperatures just a few degrees above absolute zero. To achieve this frosty temperature, researchers had to cool the materials with costly liquid helium.
In 1986, researchers discovered high-temperature superconductors that operated at subzero temperatures accessible using relatively cheap liquid nitrogen. Still, scientists wanted more convenient superconductors that ideally did not demand any unwieldy, energy-sucking refrigeration.
The most recent breakthrough arrived in 2020, when Dias and his colleagues reported the first evidence of room-temperature superconductivity at roughly 59 degrees Fahrenheit. But this historic effort required pressures of 267 gigapascals — more than 2.6 million times atmospheric pressure. So it wasn’t exactly ready for MRI machines in hospitals near you.
Keeping it room temp
In a new study, researchers from the University of Rochester say they’ve concocted a superconductor that requires relatively low pressures and moderate temperatures.
In the new study, Dias and his colleagues say their room-temperature superconductor can offer superconductivity at 69.5 degrees Fahrenheit and just 1 gigapascal of pressure. That’s still an extraordinary amount of pressure — more than the pressure at the bottom of the Mariana Trench, the deepest point in the ocean — but microchip fabrication techniques, for example, regularly incorporate materials held together by even greater internal pressures.
"This is a very significant development, akin to the transition from the horse-drawn buggy as a means of transportation to driving a Ferrari," Dias says. "We are at the dawn of a new century that will be enhanced by superconductivity technology."
His team created the new superconductor by placing a sample of the metal lutetium in a reaction chamber with a gas mixture of 99 percent hydrogen and 1 percent nitrogen. Then, like a tasty stew, they let the combination cook at high temperatures for a few days.
Electrons in superconductors no longer repel each other, as they do in most materials. This means they can form pairs and withstand the resistance they would ordinarily experience from atomic nuclei as they move about.
These electrons often couple together due to vibrations in the superconductors called phonons. In the team’s new superconductor, the lutetium makes it easier for the phonons in the material to form electron pairs at lower temperatures, Dias says.
Initially, Dias envisioned metallic hydrogen as an ideal room-temperature superconductor. But hydrogen likely only solidifies into a metal form at pressures as high as nearly 500 gigapascals, so it’s tricky to generate.
This led to the team to explore compounds loaded in hydrogen as possible superconductors — they speculate that the elements in these compounds may create stable cages that could compress the hydrogen atoms, helping superconductivity occur at pressures lower than those required with metallic hydrogen.
"I am both surprised and excited by the finding of near room-pressure superconductivity," Eva Zurek, a theoretical chemist at the State University of New York at Buffalo who wasn’t involved in the new study, tells Inverse. "We have learned how to find high-temperature superconductors in the last years, but only at very high pressures. If correct, this work would give us a pathway towards that holy grail."
Conducting controversy
This new paper follows a trail of controversy: The journal Nature retracted the first room-temperature superconductor study from Dias and his colleagues last year due to concerns about its data. The researchers have resubmitted the study with new data they say validates the earlier work, findings they collected in front of an audience of scientists at the Argonne and Brookhaven National Laboratories for transparency.
To head off criticism toward the new study, Dias’ lab used a similar approach.
"We welcome the scientific community's efforts to replicate our work," Dias says.
There’s a key difference between the two papers: Dias’ first room-temperature superconductor study analyzed a mix of carbon, hydrogen, and sulfur, but the new study mentions a combination of lutetium, hydrogen, and nitrogen.
When it comes to the former, other labs haven’t been able to find the precise ratios that could lead to a room-temperature superconductor. And as for the latter, "I cannot see why lutetium hydride would be a high-temperature superconductor at all," Artem Oganov, a crystallographer at the Skolkovo Institute of Science and Technology in Moscow, who did not take part in this research, tells Inverse. "These results will need a careful check by the community."
One major obstacle confronting all high-pressure superconductor research: It’s difficult to create and study these special materials. For example, it’s hard to run the electrical and magnetic tests needed that show whether these materials work as superconductors or not. And scientists often don’t even know the exact ratios of the elements after cooking them.
If future research confirms this new superconductor is the real deal, scientists like Dias can then aim to discover its specific concentrations of lutetium, hydrogen, and nitrogen, as well as the position of these atoms within its structure. This may help demystify its superconducting state.
Another exciting possibility: training machine-learning software on the data from their superconductor experiments to predict other possible superconductors, Dias says.