Solar wind study explains why the northern lights are so spectacular
This may also explain why there are more blackouts in the Northern Hemisphere.
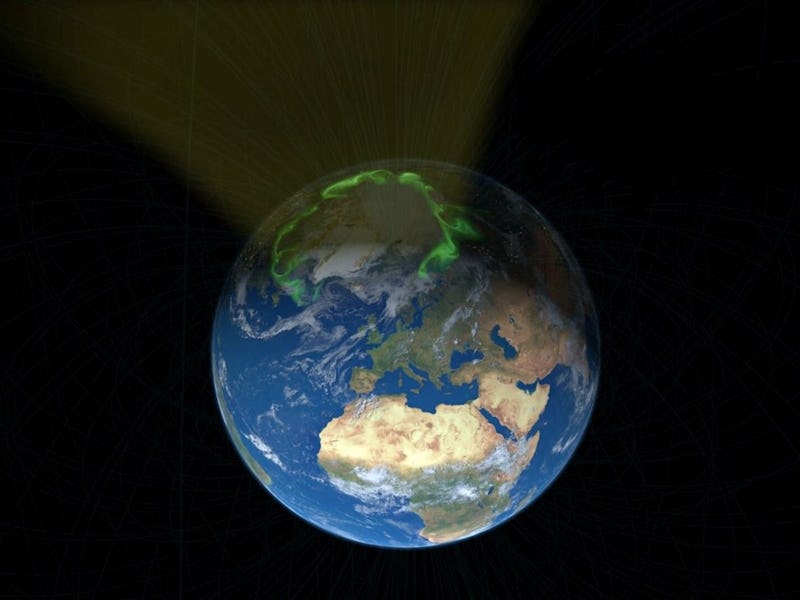
Throughout its 11-year cycle, the Sun occasionally sends explosive streams of charged particles our way. As they travel towards Earth, these particles interact with our planet's atmosphere. In the aftermath of the collision, a beautiful display of green, purple, and red lights may twist across the sky.
Near the Earth's North Pole, these lights are known as aurora borealis, or the famous northern lights. At the South Pole, the same process is called the aurora australis, or the less famous southern lights. In a new study, astronomers reveal the reason why the northern lights may be so much better known than their southern counterparts may be to do with the Sun itself.
What they discovered — In a new study published this week in the journal Nature, a team of scientists show how solar wind emitted by the Sun favors the Earth's magnetic North Pole rather than the South Pole. In other words, space weather may be worse in the Northern Hemisphere than in the south.
The findings have implications for how space weather affects us down here on Earth, with the Northern Hemisphere being subjected to harsher solar winds — and more potential damage.
Here's the background — Solar activity largely depends on the Sun's magnetic field.
The Sun’s magnetic field goes through a periodic cycle in which the south and north poles essentially switch spots, and it takes another 11 years or so for them to switch back. We're currently in Solar Cycle 25.
Solar flares are intense, bright bursts of radiation linked to the star's magnetic energy. As these flareups shoot out into space, lots of that energy, in the form of streams of charged particles, travels towards Earth.
Space weather is controlled by the flareups of the Sun that are ejected into outer space.
Earth's magnetic field shields us from most of these particles — like an umbrella protects from Earth's bad weather, so too does our magnetic field fend off the ill-affects of solar wind. But this shield is only so strong, and sometime the charged particles can travel along our planet's magnetic field lines at the North and South Poles and seep into Earth’s atmosphere.
As the particles interact with the different gases found in our planet's atmosphere, namely oxygen and nitrogen, the atoms are excited by the interaction and emit light. This process is what causes the auroras that we see at the North and South Poles
The sun's charged particles interact with Earth's atmosphere to create this beautiful display of glowing light.
What's new — Scientists had assumed solar wind travels evenly to both the Southern and Northern Hemispheres, with an even distribution of electromagnetic radiation to the North and South Poles.
But the new study suggests solar wind tends to favor the north, with more charged particles traveling towards the Northern Hemisphere.
The team of scientists behind the new study believe that this asymmetry may be caused by the fact that Earth's magnetic South Pole is further away from Earth’s spin axis than the North Pole, affecting how much energy makes its way towards the Northern and Southern Hemispheres.
“We are not yet sure what the effects of this asymmetry might be, but it could also indicate a possible asymmetry in space weather and perhaps also between the aurora australis in the south and the aurora borealis in the north," Ivan Pakhotin, a researcher at University of Alberta's physics department and lead author behind the new study, said in a statement.
How they did it — The discovery was made using the European Space Agency's Swarm satellites, a group of three identical satellites named Alpha, Bravo, and Charlie that launched into space in November, 2013 to provide precise measurements of Earth's magnetic field and interactions in the planet's atmosphere.
Why it matters — Space weather is no joke. Coronal mass ejections are highly energetic eruptions from the Sun and the main source of major space weather events.
Essentially, these eruptions are giant bubbles of gas and magnetic flux released from the Sun with up to a billion tons of charged particles, traveling at high speeds that can reach several million miles per hour. These clouds, and the shock waves they cause, can sometimes reach Earth and cause geomagnetic storms.
These storms, if they are especially powerful, can wreak havoc on the electronics within satellites, pose a threat to astronauts with their radiation, and disrupt power grids on Earth enough to black-out a city.
Back in 1859, an outburst of plasma from the Sun's corona, now known as the Carrington Event, knocked out telegraph systems all over the world. As some telegraph operators tried to send and receive messages, they received electric shocks.
A coronal mass ejection on Feb. 27, 2000 taken by SOHO LASCO C2 and C3. A CME blasts into space a billion tons of particles traveling millions of miles an hour.
What's next — Scientists are working towards being able to better forecast space weather so that they can predict when these powerful solar storms may occur.
“The Sun’s activity, such as mass coronal ejections, can have potentially serious consequences for our modern way of living," Ian Mann, a researcher from the University of Alberta, and co-author of the study, said in a statement.
"Studying the underlying physics of space weather and the complexities of our magnetic field is very important to building up early warning systems and designing electrical grids better able to withstand the disturbances the Sun throws at us," Mann says.
Scientists will need to investigate the Sun's activity further in order to find out how much this asymmetrical distribution of its electromagnetic radiation affects our planet, and whether the Northern Hemisphere may be more vulnerable to damage during a solar storm than the Southern Hemisphere.
Abstract: Terrestrial space weather involves the transfer of energy and momentum from the solar wind into geospace. Despite recently discovered seasonal asymmetries between auroral forms and the intensity of emissions between northern and southern hemispheres, seasonally averaged energy input into the ionosphere is still generally considered to be symmetric. Here we show, using Swarm satellite data, a preference for electromagnetic energy input at 450 km altitude into the northern hemisphere, on both the dayside and the nightside, when averaged over season. We propose that this is explained by the offset of the magnetic dipole away from Earth’s center. This introduces a larger separation between the magnetic pole and rotation axis in the south, creating different relative solar illumination of northern and southern auroral zones, resulting in changes to the strength of reflection of incident Alfvén waves from the ionosphere. Our study reveals an important asymmetry in seasonally averaged electromagnetic energy input to the atmosphere. Based on observed lower Poynting flux on the nightside this asymmetry may also exist for auroral emissions. Similar offsets may drive asymmetric energy input, and potentially aurora, on other planets.