Groundbreaking new measurements confirm one of Einstein's most mind-bending theories
NIST scientists are one step closer to uniting quantum mechanics with gravity.
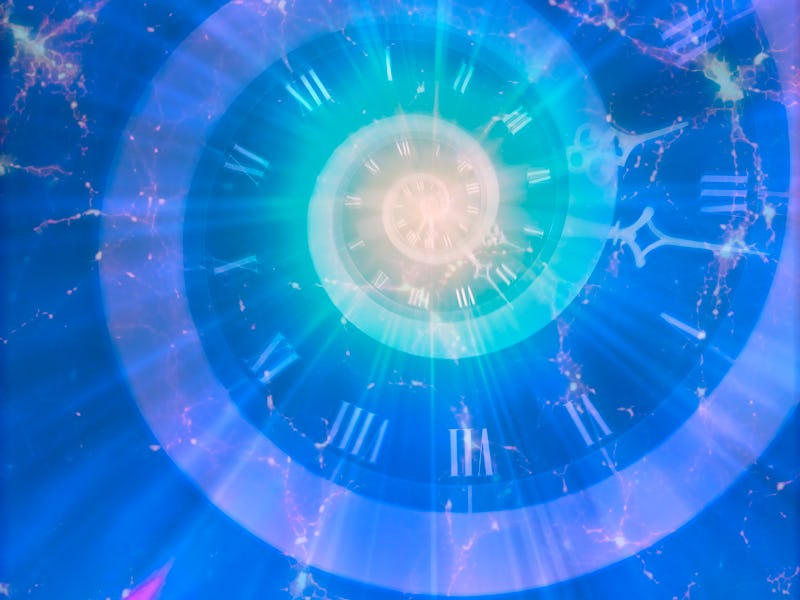
When it comes to testing a scientific hypothesis, some disciplines have it easier than others. In a biology lab, for example, you might have living cells to poke and prod, or in a chemistry lab, you may be able to clearly watch the reaction of chemical compounds. But for physicists studying unseen phenomena like gravity and time, proving scientific predictions can be a little more challenging.
In particular, physicists have a storied history of going to — literally — great lengths to test Albert Einstein’s theory of general relativity through the decoherent ticking of incredibly accurate atomic clocks. Atomic clocks have been brought to the tops of tall buildings, flown in airplanes, and even boarded spacecraft in search of answers. Now, researchers from the JILA research institute have presented the most precise measurement to date for this question on the scale of 1 millimeter in a paper published Wednesday in the journal Nature.
Toby Bothwell is first author on the paper and a graduate student at the University of Colorado, Boulder. He tells Inverse that this new measurement was made on the backs of decades of existing research.
“In 2010 researchers at NIST [National Institute of Standards & Technology] compared two aluminum ion clocks separated vertically by 30 centimeters, the smallest distance before this result,” Bothwell says. “We built a new experimental apparatus using the latest results from decades of advances … that allowed us to measure time dilation at a scale of around 200 microns, not much larger than a human hair — and a factor of almost 1000 times smaller than the previous best result.”
Here’s the background — From the human perspective, time is one of the only currencies we cannot manipulate. We grow older at the same rate every day and time moves at the same rate for all living creatures — as the grating saying goes, we all have the same hours in the day as Beyonce.
Except, according to a 1915 prediction by Einstein, this may actually be fundamentally false.
According to his general theory of relativity, Einstein postulates that gravity can actually affect the flow of time and that objects further away from the Earth’s gravitational pull (e.g. an atomic clock in an airplane) should “experience” the passing of time at a small but noticeably different rate. This idea that the experience of time isn’t fixed is called “time dilation”.
For scientists like Bothwell, this time dilation effect can be measured using extremely precise atomic clocks and can help not only further confirm Einstein’s theory but reveal more about the relationship between quantum mechanics and gravity as well.
Why it matters — Despite being a force we experience daily, gravity is actually one of the fundamental forces that plays least well with other forces in our universe, including electromagnetism and nuclear forces. Famously, gravity and quantum mechanics do not mix and represent a giant gap between our understanding of the macro world of humans and the quantum-governed micro world of particles.
Jun Ye is a coauthor on the paper and principal investigator of the JILA research group. He says that future atomic experiments on this scale or smaller could be an important step toward understanding how gravitation works on the small scale.
“In the microscopic world of quantum, atoms are sometimes represented by quantum waves [and] quantum wavelength[s] can extend over a scale of a few microns,” Ye tells Inverse. “We want our clock to gain the sensitivity such that over this wavelength scale it can tell the time is evolving differently, all due to the presence of a very macroscopic body of our mother Earth.”
Additionally, Bothwell says that more precise measurements of this dilation also have a practical purpose as well when it comes to correcting accumulating GPS errors created by drifting clocks and even one day serving as an early alert system for volcanic eruptions by detecting small changes in elevation of the Earth’s crust.
The stronium clock at NIST used in the experiment.
What they did — To make these measurements, the team looked at 100,000 ultracold Strontium atoms in their lab that were trapped within an optical lattice, which Ye describes in a video about the experiment as akin to a stack of pancakes.
The team then split this pile of “pancake” atoms and separated them by a distance of 1 millimeter. They report that the atoms were able to maintain their shared energy state for 37 seconds before the effects of time dilation took hold and ever so slightly nudged the clocks out of alignment. With over 90-hours of data, the team was able to measure this slight decoherence with 50 times more accuracy than previous atomic clock comparisons.
What’s next — Going forward, Bothwell says that the team is excited to work towards shortening the distance between their lattice clocks even further. Through this work, he hopes to inch closer and closer to understanding the very nature of space-time itself.
“We have a long way to go before such clocks can be sensitive to the curvature of space-time and ask questions about the connection of gravity and quantum mechanics,” Bothwell says. “[But] with improved lasers we can measure the time dilation below the 100 micron scale, continuing to march towards sensitivity to space-time curvature.”
Abstract — Einstein’s theory of general relativity states that clocks at different gravitational potentials tick at different rates relative to lab coordinates—an effect known as the gravitational redshift. As fundamental probes of space and time, atomic clocks have long served to test this prediction at distance scales from 30 centimetres to thousands of kilometres. Ultimately, clocks will enable the study of the union of general relativity and quantum mechanics once they become sensitive to the finite wavefunction of quantum objects oscillating in curved space-time. Towards this regime, we measure a linear frequency gradient consistent with the gravitational redshift within a single millimetre-scale sample of ultracold strontium. Our result is enabled by improving the fractional frequency measurement uncertainty by more than a factor of 10, now reaching 7.6 × 10−21. This heralds a new regime of clock operation necessitating intra-sample corrections for gravitational perturbations.
This article was originally published on