Scientists Prove Decades-Old Theory That May Change Particle Physics
We're finally cracking the code of elusive "neutrinos."
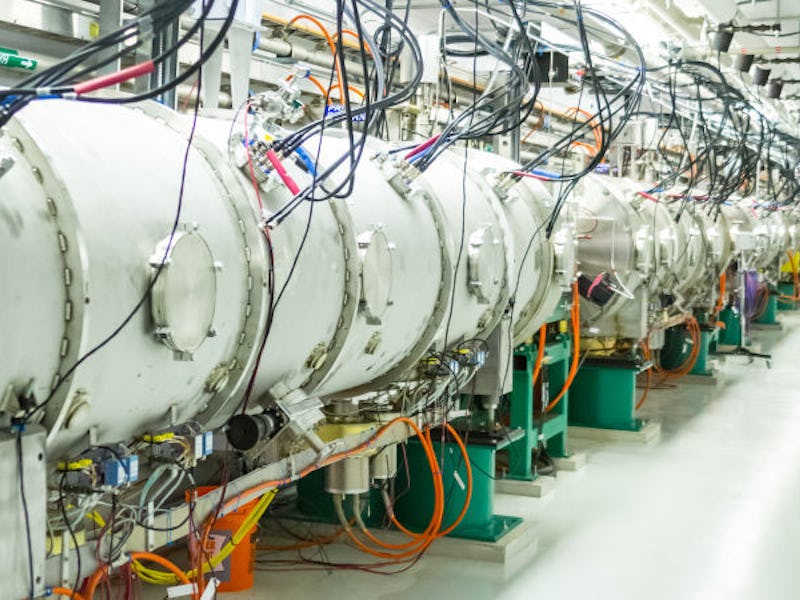
Neutrinos are among the most mysterious particles in existence. They’re extremely hard to detect because they tend to shun other particles, but we do know that they’re blasted out by supernovae, that one hundred trillion shoot through our bodies every second at almost the speed of light, and that they might be similar to dark matter.
Now we know a bit more: Scientists at the Department of Energy’s Oak Ridge National Laboratory have just proven a decades-old theory about neutrinos that has huge implications for astrophysics and even the “Standard Model” of particle physics.
Until now, we could only find neutrinos by spotting their interactions with protons. Such a collision causes the proton to decay into a neutron and an electron and that electron to create a small flash of measurable light.
Now we have a new detection method — though in a way it’s not new at all, because physicist Daniel Freedman theorized about it in a 1974 paper. He suspected that neutrinos interact with atomic nuclei as well as protons. Forty years later, Freedman has finally been proven right.
The Oak Ridge lab used a machine called the Spallation Neutron Source (SNS) to shoot neutrons down a beam line, a process that creates neutrinos as byproducts. They placed the SNS in front of a 20-foot-thick concrete wall that would block most particles but allow neutrinos to pass through.
SNS’s Beamline 13.
“Because SNS produces pulsed neutron beams, the neutrinos are also pulsed, enabling easy separation of signal from background,” reads the press release. The physicists used these pulses to detect the neutrinos hitting atomic nuclei, just like Freedman postulated they would.
This tactic for finding neutrinos is much better than the old: The odds of neutrinos interacting with nuclei are 100 times greater than the odds of them interacting with protons, simply because nuclei are much bigger than protons.
What’s the significance of all this? It means that physicists can create neutrino detectors that are a much smaller, handheld size. This — along with the fact that we now know that neutrinos can, indeed, interact with nuclei — has even bigger implications. In fact, we’ve just taken a major step toward better understanding supernovae and finally identifying dark matter.
Dark matter is even more difficult to detect than neutrinos (so much so that we’re still not even sure what it is). Some scientists think that dark matter particles are actually quite similar to neutrinos: that they also interact with nuclei and could be found by observing the reactions of those nuclei. If that’s true, physicists will need to find a way to filter out the signals of neutrinos colliding with nuclei in order to hone in on dark matter collisions. “Yesterday’s signal becomes today’s background noise,” physicist Hirohisa Tanaka told WIRED. Now that scientists know more about neutrinos, they have more of an idea of what they’ll need to filter out.
A ring of dark matter that the Hubble telescope detected in 2007.
Supernovae — the expanding balls of gas that erupt when massive stars explode — are better understood than dark matter, but they’re still enigmatic. Astronomers have modeled ways that these events may begin and unfold; more information about neutrinos will help corroborate or disprove those models, because 99 percent of supernovae energy is emitted as neutrinos.
“When a massive star collapses and then explodes, the neutrinos dump vast energy into the stellar envelope,” COHERENT’s spokesperson, Kate Scholberg, said in the press release. “Understanding the process feeds into understanding of how these dramatic events occur.”
This new method for finding neutrinos could even help scientists work out some of the kinks in what’s known as the “Standard Model” of particle physics. The model seems to fit most phenomena, but physicists have found that it doesn’t quite fit neutrinos; according to the model, neutrinos shouldn’t have any mass — but they do. Now that there’s an easier, smaller, and lighter way of detecting neutrinos, physicists may finally be able to figure out what in the model is wrong.
As if all of that wasn’t enough, scientists may be able to use this new method “to better understand the structure of the nucleus,” Scholberg said in the press release.
The implications are so wide-ranging that they’re difficult to even comprehend, but we’ll see them play out in the coming years as physicists learn even more about neutrinos and use them to unlock the mysteries of our world and outer space.