Don't Expect a Quantum Smartphone Anytime Soon
But quantum computing could change biochemistry, materials science, and encryption.
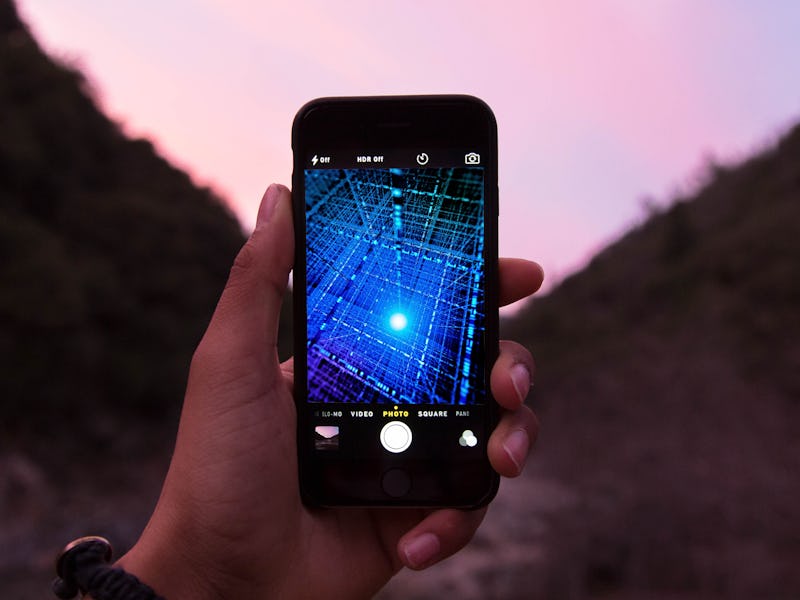
Quantum computers have acquired an almost mythical quality for parts of the sci-fi and tech-focused internet because we hope they end up being scientific tools for solving some of the most difficult and important problems in the universe.
By building a working understanding of the incredible new physics of “quantum bits,” scientists are building the tools that could help design a clean form of mass energy production, or even new medical therapies.
A quantum computer is the sort of thing that most people wouldn’t believe if they heard about it in a bar. It’s defined as a computer “which makes use of the quantum states of subatomic particles to store information,” according to the Oxford Living Dictionary, but it’s actually much more complex than even that.
Rather than computing with bits of information that are in either a 1-state or a 0-state, quantum computers compute with qubits of information (pronounced “KYOO-bits”) that are simultaneously in a 1-state and a 0-state thanks to the magic of quantum physics. In fact, qubits must be in this nonspecific state of “superposition” or the quantum computer won’t function. Keeping the qubits in this fragile state requires shielding the computer from any interaction with the outside environment, including observation by the operators of the computer itself, meaning that quantum computers are black boxes. Inputs enter, travel every possible path through the qubits simultaneously, and then an output is produced. Just don’t look inside, though — if you do, the whole thing stops working.
They are keeping up to 2,000 separate particles in quantum superposition, and if you believe some people, coordinating calculations on those particles across parallel universes.
As insane as that sounds, quantum computers really do exist in the world right now. Canadian startup D-Wave Systems has famously sold specialized quantum computers to everyone from Google to the U.S. government, and since Lockheed engineers don’t tend to buy mutli-million dollar computers that don’t work, the news has sparked renewed interest in the seemingly exaggerated statements about the technology’s potential. D-Wave’s machines still can’t be used to run all forms of quantum algorithm, but they could offer enormous speed advantages in specialized fields.
It’s unlikely the world will see a quantum smartphone anytime soon, but the technology’s applications to biochemistry, materials science, and encryption could change the world far more than any consumer product.
The reason for the incredible disruptive potential, as pointed out by Dave Wecker of Microsoft’s LIQUi|> (pronounced “liquid”) or Language Integrated Quantum Operations, is that quantum computers are best suited to different sorts of problems. Among other things, LIQUi|> can simulate a quantum environment so would-be quantum programmers can test their code.
“The main thing a quantum computer does is open up new areas that classical machines really can’t address,” Wecker tells Inverse. “Quantum computers are not a threat to classical machines.”
Consider the case of a 10-bit computer and a 10-qubit quantum computer. Digital computers have 2n possible states, where n is the number of transistors in the processor, meaning that a 10-bit computer can have 1024, or 210, different combinations of 1’s and 0’s. Since regular computers follow regular logic, it will thus take 1024 cycles of the processor to check every one of these 1,024 possibilities. A 10-qubit quantum computer on the other hand, keeps its 10-qubits in a superposition of states, meaning that it can check all 1,024 possibilities at the same time. In this case, that makes the quantum approach about a thousand times faster at the very specific task of checking raw combinatorial possibilities, and the speed differential only goes up as the quantum processors incorporate more qubits.
There are real applications for that sort of speed, today. The furthest along is probably analysis of protein folding, a problem so computationally difficult that scientists have asked the entire world to donate computer time. The question is just how a linear series of amino acids, specified by a gene, folds up over and over inside a living cell to create the complex three-dimensional structure of the final protein, which then dictates that protein’s behavior. Quantum computers have already shown great promise in battering through the sheer scale of the challenge of figuring out the rules that govern this process, and if they’re successful they could spark an unprecedented revolution in medicine.
Additionally, Wecker pointed out that currently, around 3 percent of the world’s energy goes into the production of fertilizer to keep food growing, but the “Haber process” of fixing nitrogen in these fertilizers is far less efficient than the system used by natural, nitrifying bacteria that do much the same thing far more slowly. By applying the sheer power of quantum computers to the bacterial proteins of this nitrification process, quantum could let agriculture take the biggest step forward since the First World War, not only improving food production per acre but greatly reduce emissions from the industrial processes. The ability to study the exact structure and function of proteins could also reveal the exact workings of cellular photosynthesis, which is also far more efficient than today’s solar photovoltaic cells, increasing solar energy output and potentially making the process carbon-fixing as well.
Once we’ve used quantum computers to design these artificial leaves to make all that nice free solar electricity, though, what are we to do with it? Today, there are only three answers: use it right away, store it at a great cost, or lose it as though it never existed at all.
Many scientists hope that quantum computers could help with design of so-called high-temperature superconductors, which can conduct electricity with literally zero resistance, and thus zero loss of energy during transmission. There are already some short superconducting grid cables, but they’re much too expensive to revolutionize the global grid, as a whole.
Thus, we can realistically imagine quantum computers being used to study proteins, allowing the collection of nearly unlimited solar energy on the sands of the Sahara Desert, and then being used to study materials, allowing that energy to be sold in Reykjavik, or Moscow, or Orange County.
But revolutionizing medicine and power production is just the beginning — quantum computers could be the most disruptive technology to national security since nuclear weapons. The reason is that virtually all of modern security is ultimately based on the simple computational difficulty of factoring large numbers. This is the mathematical shield that protects everything from Facebook messages to the U.S. power grid, and quantum computers are perfectly suited to blowing past it.
The National Security Agency headquarters in Fort Meade, Maryland.
That’s why quantum computers are rumored to be at the heart of secret government surveillance plans that can read the unprotected communications of everyone — because someday, they really could be. A factoring problem that might take a modern computer centuries to complete could theoretically be solved by a quantum computer in a matter of seconds.
Google's campus.
Right now, the most advanced quantum computers are coming from Google and IBM, but they don’t incorporate enough qubits to be used for this purpose. On the other hand, D-Wave’s 2000 qubit computer is more than big enough, but it uses a more limited approach called quantum annealing, which can’t execute the factoring algorithm that could end all of modern computer security. This means that it’s unlikely the NSA has really managed to use quantum computers for that purpose, but it’s a foregone conclusion that they’re trying to develop annealing algorithms that could be.
If the FBI had had a working quantum code-breaking machine during and after its infamous Apple encryption showdown, negotiation would have been totally unnecessary, and there wouldn’t have been a thing Apple could have done to stop the FBI from taking whatever it liked. Quantum computers have such enormous potential to break encryption that just the possibility of quantum machines has led to the formation of an all-new area of mathematics called “post-quantum cryptography,” focused on finding algorithms efficient enough to be used every day that could nonetheless offer protection against the power of a quantum machine.
D-Wave’s newest model of limited quantum computer goes for a cool $15 million, however, and with price-tags like that there just aren’t a lot of units available to study. Thankfully, IBM is making access to its IBM Q prototype available over the cloud, and Wecker’s own LIQUi|> project features a quantum computer simulator that allows researchers to run their code, though only at normal speeds. It doesn’t provide the incredible speed benefits of a real quantum computer, but it does let you check to see if your code will work on one.
Remember, whenever hype for quantum computers crosses from their problem-solving applications to their potential in consumer electronics: the most powerful quantum computer in the world today can’t even run a copy of Pong. The potential speed of a quantum computer could be literally unimaginable — but only where it best applies.
All this means that, even in the mid- and long-term future, quantum computers might not replace the processor in your phone, not even through the cloud. Rather, Wecker envisions a role not unlike that for GPUs. “Since the quantum computer will most likely be a co-processor, it’s probable that the O/S will really reside on the classical machine that runs it.” What will be key is developing the breadth of software necessary to fully exploit this hybrid environment.
“This is why we made [LIQUi|>] available to everyone. We’re hoping that lots of people getting a chance to program a quantum computer (even in simulation) will lead to breakthroughs in fundamental algorithms.”
Much like the current rise of neuromorphic computers, the coming rise of quantum computing won’t so much supplant existing computers as supplement them. Neuromorphic computers will take some of the jobs that tax classical computers, like machine learning, while quantum computers will take others, like mass sorting and brute force possibility checking. This leaves classical computers to do the things they do best: linear math, and the execution of ordered, unchanging commands. They could even find a permanent place running slow, limited, but above-all transparent simulations of quantum computers, letting coders openly check their algorithms before they enter the quantum box.
So, leave the conspiracy theories at the door — they really aren’t necessary to find a truly bizarre and exciting area of research. We might never get a quantum-speed game engine, or even a Windows Q, but if quantum computers can solve some of the most pressing problems in science and engineering today, they could change the world far more fundamentally than that.